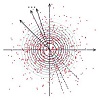
My two latest papers[1,2] offer a simple and natural resolution to some of the most profound questions of particle physics and cosmology.
Skepticism about having found
as early as in 2012
the correct answers to "most of the problems of modern physics"
— which is how an eminent US physicist characterized
the questions answered —
is understandable and healthy. Nevertheless, please consider that:
My education and qualifications
are well suited to explore the areas addressed.
My previous work in theoretical particle physics and cosmology
has consistently yielded acknowledged
findings
about our real physical world.
Some of them have already been confirmed experimentally.
The derivation of the current results has been written
up in detail. They form a closed and natural picture that is remarkably straightforward.
The quantum properties of our world,
which are still puzzling to essentially
everyone else, from this perspective
are utmost natural and simple. So is natural and simple their origin.
Finally, with the general picture available
since 2012 and with many opportunities to reexamine it, the obtained results have been verified
and understood thoroughly in several equivalent descriptions.
Some of the fundamental questions answered in [1] and [2] are:
- Do the laws of physics — including the postulates of quantum mechanics, quantum field theory, and general relativity — arise naturally from something much more elementary?
- Why does physical evolution proceed by specific, unchanging fundamental laws?
- What laws govern evolution at the shortest physical scales (at the Planck length), where the Standard Model of particle physics and canonically quantized general relativity[3] break down?
- Why do time and space unify in classical relativity but differ conceptually in quantum physics, even of relativistic systems? Why is the observed physical dynamics local? Why are its laws highly symmetric, e.g., have gauge symmetries, leading to the electromagnetic, weak and strong interactions, and have general covariance, producing gravity?
- Which of the various "interpretations" of quantum mechanics (e.g., Copenhagen, many-worlds, hidden-variable, stochastic, super-deterministic, etc.) — some of which are different physical theories, predicting different outcomes of feasible experiments — is the correct one?
- Why do the observed large-scale cosmic structure and fluctuations in the cosmic microwave background indicate that our universe has undergone cosmological inflation, despite convincing theoretical arguments from esteemed physicists [4, 5, 6, 7, 8, 9] that it is more probable to live in anthropically suitable matter configurations that have never inflated, e.g., in Boltzmann-brain worlds[10, 11]?
- How to reconcile the unitarity of quantum evolution with the apparent loss of information during the Hawking evaporation of a black hole? How does its evaporation end? What does an observer who falls in a black hole, see at its horizon? What physics applies to the singularity at its center?
The fundamentals of the physical world appear to be surprisingly simple. Their understanding does not require abstract mathematical or philosophical concepts. The only material structure behind the quantum-field worlds identified in [1] is the general distribution dN/(dQ1dQ2...) of a large number N of any material entities over many of their arbitrary independent properties Q1, Q2, ... that can be quantified with real numbers.
The nature of the material entities that form the base of the emergent worlds and are characterized by the quantities Q1, Q2, ... is irrelevant. If our universe originates as described, its material base is all objectively existing information carriers, not limited to objects in our or other emergent physical universes. The most basic material entities are not expected to be embedded in any external spacetime or to evolve. It is sufficient for them just "to be".
The emerging physical worlds at sub-Planckian energies are succinctly described by effective quantum field theory, with all its standard mathematical machinery. The fields of the elementary particles of the worlds undergo well-defined evolution, tractable at every physical scale.
The wave function of the emergent fields consists of alternate, equivalent presentations of the smoothly coarse-grained general distribution dN/(dQ1dQ2...) of the arbitrary basic material entities over their general properties Qn, [1]. The elementary particles of the respective emergent physical worlds are quantized excitations of the fields. The general relativistic, quasiclassical spacetime of the worlds arises as detailed in [1].
The Born rule for the probabilities of alternate outcomes of quantum processes in the emergent worlds is not a postulate. It arises dynamically from first principles. The worlds evolve naturally from cosmological inflation.
Unlike previous theoretical approaches, we do not postulate fundamental physical principles, dynamics, or initial conditions. They all are what they are for the generically emerging quantum-field worlds, which actually and objectively exist in nature. The dynamics and initial conditions for a general class of the emergent systems are, as currently understood, apparently indistinguishable from those observed in our universe.
This fundamental picture of quantum evolution is a middle ground between the interpretations of quantum mechanics that suggest only one branch of macroscopic evolution and the Everett interpretation (where each of the alternative outcomes of quantum evolution predicted by standard quantum theory with non-zero probability is assumed to be real and physically co-existing “in parallel” with the other outcomes, however minuscule the norm of the respective branch of the wave function is). Ditto the popular today — and vigorously explored by physicists and sci-fi writers for the past half-century — multiverse paradigm. The described picture permits a variety of non-communicating universes with different physical laws, perhaps justifying anthropic explanation[12] of the tiny but non-zero cosmological constant. Yet, by this picture, the laws of the physically real emergent universes span an infinitesimal subset of all conceivable anthropically suitable laws of dynamics. Notably, this understanding of fundamentals offers concrete answers[2] to notoriously difficult questions about black holes: their information paradox, the physics at their horizon and center, and the final moments of their Hawking evaporation.
The structure that produces the emergent quantum-field worlds described is extremely general. In particular, it is ubiquitous in our familiar physical world. Thus, these emergent worlds certainly exist in nature. They evolve by dynamics and from initial conditions that meet the strict constraints imposed by particle-physics experiments, cosmological observations, and other empirical data present. Some of these worlds are apparently indistinguishable from our observed universe. They contain internal inhabitants like us. Some of their inhabitants are being occupied with what we currently are. Some, in particular, are pondering if their world originated as it did. It may be presumptuous to insist that we are not them. After all, their universe — physically real in every sense — is one of the most typical objectively existing physical worlds regardless of what the basic constituents of nature are.